
It’s that time of the year again when I’m tasked to write another article for our Foliar-Pak website. I used to write quite a bit in a previous life, but sadly my day-to-day requirements don’t call for many well-written tomes—unless you count hundreds of emails (which are probably neither well written nor incredibly tome-like). Luckily, we here at Foliar-Pak have an outstanding marketing team, and they’re kind enough to feed us ideas about which we can write. For this particular article, the marketing team thought that writing about each nutrient’s role in plant development would be a great idea. In my sleep-deprived and over-worked state, I heartily agreed—that is until it came time to write this. For those in the know, plant nutrition can be a very complex topic, especially when you start to break down each nutrient’s function and role within plants. There have been countless books written on the subject and none of them are what I’d call a “quick read.” Realizing this, I’d like to offer a “CliffsNotes” version of several of these texts. A cheat sheet of sorts that you can refer back to again and again. As Albert Einstein once said, “why memorize something when you can look it up?” In less than 2000 words, I’d like to provide the information you need to know while saving you valuable time to do other things you may enjoy in life. A simple thanks via social media would be great!
[Author’s note: All of the following information came from a variety of peer-reviewed, published works. To save time, let’s just assume that all of the following scientific information came from one of those sources and not directly from me. All editorial comments (you’ll know the difference) are my own.]
NITROGEN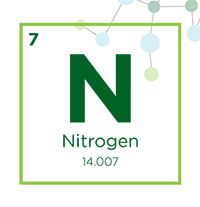
The essential role of nitrogen is as a constituent of amino acids in plants—also known as the building blocks of proteins. Amino acids are assembled into peptides (small chains of amino acids) and proteins (large chains of amino acids). Proteins serve a wide range of functions including structure, movement, storage, and transport. Aside from peptides and proteins, nitrogen is found in a variety of compounds, including but not limited to purines, alkaloids, enzymes, vitamins, hormones, nucleic acids, and nucleotides.
Conclusion: Nitrogen is pretty darn essential in plants.
Fun fact: ~85% of nitrogen in plants is sequestered in protein; ~10% in nucleic acids; and ~5% in the soluble metabolic pool of amino acids.
PHOSPHORUS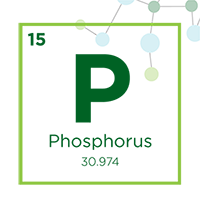
Most of the phosphorus in plants is found in ATP (also ADP and AMP), nucleoproteins, and phospholipids. ATP is an organic compound that provides energy for many different metabolic processes in plants. Nucleoproteins are any proteins that are structurally associated with nucleic acids; examples include ribosomes and nucleosomes. Finally, phospholipids (lipids that contain phosphorus) are a structural double-layer component of cell membranes.
Conclusion: Energy doesn’t get stored, and cell walls don’t get built without dear old phosphorus.
Fun fact: The form of phosphate matters. Plants need phosphorus in the form of phosphate (PO43-) to be usable in the ways listed above. Phosphorus in the form of phosphite (PO33-) does not convert to phosphate in the plant.
POTASSIUM
Potassium’s primary function in the plant centers around its role as an osmolyte—a substance that is loaded into the vacuole, thereby activating the regulation of osmotic pressure and maintaining the homeostasis of a cell’s water content. This directly/indirectly affects enzyme activation, protein synthesis, stomatal pore opening and closing, photosynthesis, and transport of sugars, nutrients, and amino acids.
Conclusion: Plants would have a hard time holding water without potassium.
Fun fact: Potassium is the only nutrient in the plant that never becomes part of an organic (carbon-containing) compound.
CALCIUM
Calcium is mostly used to stabilize phospholipid membranes by bridging phosphate and anion complexes located at the hydrophilic (water-loving) membrane surface. It also forms calcium pectate in the middle lamella (pectin layer that cements the cell walls of two adjoining cells together). Calcium is also involved in other processes such as cell division, callose synthesis in response to injuries (mechanical or pest), nitrogen metabolism, and root lubrication.
Conclusion: Cell walls wouldn’t hold together without the stability of calcium.
Fun fact: After potassium, calcium is typically the most abundant element in plants.
MAGNESIUM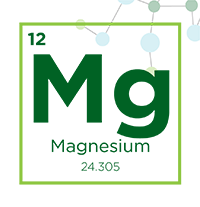
Aside from being a constituent of chlorophyll, magnesium (like calcium) is also a component of the middle lamella. In addition to this, it’s required for the preservation of ribosome structure and integrity (up to 90% or more of cellular magnesium is bound mainly in ribosomes). Magnesium also influences many different metabolic processes by complexing with anionic molecules and with negatively charged ligands.
Conclusion: Magnesium does more than just hang out in chlorophyll.
Fun fact: ATP must be bound to a magnesium ion to be biologically active.
SULFUR
Sulfur is a constituent of two amino acids: cysteine and methionine (both important to plant immune responses). It is also a constituent of several enzymes, including ferredoxin (assists in building chlorophyll and assimilating nitrogen), biotin (assists in the synthesis of fatty acids, amino acids, and the generation of glucose), and thiamine (breaks down sugars of amino acids). It is also a component of sulfolipids (specific plant membranes).
Conclusion: Sulfur is the building block for many vital enzymes and molecules in plants.
Fun fact: Sulfur is found in the thylakoid membranes of chloroplasts as sulfoquinovosyl diacylglycerol. This explains the chlorotic symptoms of plants deficient in sulfur.
BORON
Quoted as being “the least understood plant nutrient,” boron provides structural linkages within cell walls. Boron deficiencies are often associated with compromised cell membrane function. Boron forms complexes with phenolic compounds and likely provides cell membranes protection by preventing the formation of damaging toxic quinones and reactive oxygen species that arise when phenolics are oxidized.
Conclusion: You can’t build cell walls without boron.
Fun fact: Calcium-boron cross-linking at specific regions is responsible for retaining pectin polysaccharides in cell walls.
CHLORINE
Chlorine is the anion (negative charge) counterpart to the cation (positive charge) potassium. Chlorine and potassium’s roles are effectively the same in that chlorine is an osmoticum just like potassium. Chlorine is the only inorganic anion that is not structurally bound to plant metabolites. As such, chlorine serves as an anionic charge-balancing ion. Other anions such as nitrate, sulfate, and phosphate briefly serve this function but are quickly reduced (electrons added) for incorporation into proteins and metabolites. Finally, the functional assembly of a specific manganese complex and its associated proteins requires the presence of chlorine.
Conclusion: There are uses for chlorine after all!
Fun fact: The presence of chlorine in the soil can alter the production of certain root exudates and change the microbial community.
COPPER
Copper plays both important roles in protecting chloroplasts and in producing ATP. In the chloroplasts, copper is present as a copper-zinc enzyme that protects the chloroplast by transferring electrons to cell-damaging superoxide radicals, converting them into H2O2 (hydrogen peroxide). It is also a part of different enzymes that neutralize the hydrogen peroxide. In the mitochondria, it’s part of the cytochrome oxidase enzyme that helps complete the final electron transfer event required to synthesize ATP. It’s also a part of several different enzymes that are found in several different metabolic processes that assist in the breakdown of urea and the synthesis of eight different amino acids. Finally, specific copper-containing enzymes direct the synthesis of aromatic compounds required for the synthesis of lignin—an essential cell wall constituent.
Conclusion: Copper’s significance in plants cannot be understated. Have you taken a look at your copper levels within your soil, soil solution, and plant lately?
Fun fact: Copper was likely first used on plants as a fungicide in the late 19th century on grapes. Growers would mix copper sulfate and calcium hydroxide together to create what is now known as a “Bordeaux mixture.” Fungi (and bacteria) cannot regulate the uptake of copper in their cells. This leads to eventual copper toxicity and death.
IRON
Iron is required for metabolic functions related to respiration (as peroxidase, catalase, ferroxidin, and cytochrome enzymes), DNA synthesis (as a ribonuclueotide reductase enzyme), photosynthesis, and nitrogen fixation. Iron’s most well-known use involves the building of chlorophyll molecules. Much like copper, iron is not a physical part of many of the molecules in the plants. Instead, it’s mostly a part of enzymes. Chlorophyll, as an example has a molecular formula of C55H72O5N14Mg. The enzyme ferrochelatase (which does contain iron) converts protoporhyrin to heme (which does contain iron), which eventually turn into phytochromobilins that regulate the synthesis of chlorophyll. So, while iron isn’t actually a constituent of chlorophyll, it’s necessary to produce chlorophyll.
Conclusion: Iron does more than just help build chlorophyll molecules.
Fun fact: Intracelluar concentration of iron requires tight control and is regulated both at uptake and storage. In cells, most iron is stored as ferritins (iron-storage proteins). A ferritin protein can store up to 4500 atoms of iron in their central cavity. Because of this, an excess of iron increases the production of ferritin proteins in plants.
MANGANESE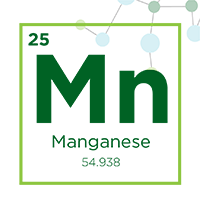
Unlike iron and copper, which are present in many different enzymes, there are only a few well-known manganese-based enzymes. Mn-SOD isoenzyme, the most well-known, operates predominantly within mitochondria to quench tissue-damaging oxygen-radicals. There is also a manganese-protein complex found in the thylakoid membranes that assist in the splitting of water molecules. Aside from these, manganese is mostly used as an activator of enzymes such as dehydrogenases, transferases, hydroxylases, and decarboxylases. Many of these enzymes are involved in carbon and nitrogen metabolism, and also play a role in secondary metabolite production such as phenolics, cyanogenic glycosides, and lignin plant defense compounds—which are all important for plant defense systems.
Conclusion: Manganese is important for plant defense, as well as maintaining the structure of thylakoid membranes.
Fun fact: Manganese can exist in three oxidative states: Mn+2 (reduced, plant-available state), Mn+3, and Mn+4. At necrotic sites of infection from fungi and bacteria, Mn+4 (plant unavailable form) typically accumulates.
MOLYBDENUM
Required in the 2nd lowest concentration than any other essential nutrient (less than 1 ppm typically), molybdenum plays an important role in nitrate metabolism in plants. Notably, nitrate reductase is a molybdenum-based enzyme that allows the plant to convert nitrate (NO3-) into nitrite (NO2-). From there the plant adds a hydrogen and creates nitrous acid (HNO2) and loads it into the chloroplast, whereby it’s eventually converted into glutamine. All of this doesn’t happen without molybdenum quietly doing its job.
Conclusion: Nitrate wouldn’t be usable in plants without molybdenum.
Fun fact: Biological fixation of N2 (by bacteria) requires enzymatic activity of nitrogenase—which involves a molybdenum-iron protein. (This isn’t directly related to molybdenum’s role in plants, but I thought it was still an interesting fact. And because there aren’t too many facts about molybdenum in plants, beggars can’t be choosers!)
NICKEL
Recognized as the 17th essential element (which includes oxygen, carbon, and hydrogen) for plant growth, nickel is a relative newcomer and is required in the lowest concentration than any other essential nutrient (less than 0.5 ppm). Nickel’s only function in plants is as a constituent in the urease enzyme. The urea enzyme catalyzes the hydrolysis of urea, forming ammonia, and carbon dioxide. While most plants only take up nitrogen through the roots as either nitrate or ammonium, there are several genera of plants that can take up nitrogen in the form of urea.
Conclusion: Nickel helps catabolize urea in plants.
Fun fact: In plants that can take up nitrogen in the form of urea, such as river birch and pecan, a nickel deficiency can cause the build-up of urea within the plant. This urea toxicity leads to the blunting of the apical tips of the foliage, causing the leaves to form the shape of mouse ears. This is why a nickel deficiency is termed “mouse ear” in plants.
ZINC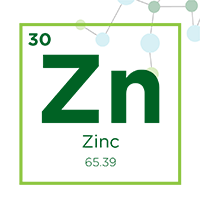
Much like iron and copper, zinc is a component of many different enzymes in plants—over 300, more than any other metal. These enzymes are responsible for a plethora of processes in plants such as (but not limited to) RNA production, hormone production (such as indole acetic acid via the synthesis of the amino acid L-tryptophan), carbon fixation (in C4 plants only), plant defense, and chlorophyll production. Zinc is also important in stomatal conductance, and a deficiency in zinc impairs the opening of stomatal pores.
Conclusion: Zinc is involved in a large amount of processes in plants.
Fun fact: Zinc is required for multiplication of every cell in plants.
But wait! There’s more! While SILICON isn’t technically listed as an “essential” nutrient in plants, it’s still important. Taken up through the roots as uncharged monosilicic acid (H4SiO4), silicon is eventually deposited as amorphous silica (SiO2·nH2O) in the cell wall matrix, cell lumen, and extracellular spaces of the shoot, leaf, culm, and root tissues. Typical benefits from an increased silica content in plants are improved canopy light interception architecture conferred by stiffer shoots (and favorably erect leaves) as well as greater tolerance to shearing and compression forces. In addition to this, silica provides a physical barrier against penetration of fungal plant pathogens and insects.
Conclusion: While plants can technically grow and survive in the absence of silicon, it can still provide many benefits to plants.
Fun fact: Silicon concentration can rival (and even exceed) Ca, Mg, and P concentrations in some plants.
Whew…there you have it, the fantastic fourteen (technically fifteen) essential nutrients and their roles in plant growth. Don’t forget to refer to this post when applying nutrition to remind you of what each component is doing to make your plants grow and flourish!